柯伊伯带小行星平运动共振研究
清华大学航天航空学院,北京 100084
THE MEAN MOTION RESONANCE OF THE KUIPER BELT OBJECTS
School of Aerospace Engineering, Tsinghua University, Beijing 100084, China
通讯作者: 1) 李俊峰,教授,主要研究方向为航天动力学与控制。E-mail:lijunf@tsinghua.edu.cn
责任编辑: 胡漫
收稿日期: 2019-04-26 网络出版日期: 2019-12-20
Received: 2019-04-26 Online: 2019-12-20
作者简介 About authors
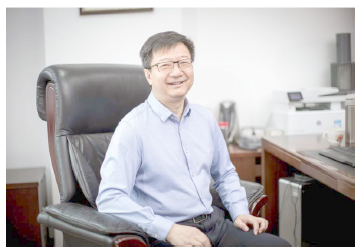
李俊峰,1987年在北京大学力学系获得理学学士学位,1993年在莫斯科大学数学力学系获得Ph.D.,1995年在清华大学工程力学系博士后出站,现任清华大学航天航空学院教授、博士生导师。获得国家科技进步奖、国家级教学名师奖、国家级教学成果一等奖,入选国家高层次人才特殊支持计划(万人计划),担任国家精品课负责人、国家级教学团队带头人,主编《理论力学》(第1、2版)被评为“十五”、“十一五”和“十二五”国家级规划教材,翻译英文教参《工程矢量力学》《理解航天》《静力学》《动力学》等,翻译俄文教参《理论力学》《力学》《理论力学习题集》等。被清华大学本科生投票评为“清韵烛光·我最喜爱的教师”,被清华大学研究生投票评为“良师益友”。中国力学学会常务理事、中国宇航学会空间控制委员会副主任、中国科学技术协会动力学与控制学科首席科普科学传播专家。
研究柯伊伯带小行星对理解太阳系的起源和演化有着重要的意义。由于距离海王星较近,柯伊伯带小行星受其影响较大。这其中,又以共振小行星和离散小行星两族受影响最为明显。本文主要讨论了这两族小行星与海王星的共振现象。共振小行星处在共振轨道中,可以避免与海王星的近距离接触,轨道相对稳定,可以长时间保持。离散小行星的演化过程是共振黏滞与引力离散共同作用的结果。本文除了综述共振小行星和离散小行星的研究进展,还进一步分析了相应共振比下共振在大偏心率时的宽度,解释了离散小行星在离散盘大量分布的原因。
关键词:
The movements of the asteroids in the Kuiper belt are important for us to understand the origin and the evolution of the solar system. Since the Kuiper belt objects (KBO) are a short distance to the Neptune of a great mass, the KBOs are significantly perturbed by Neptune's gravity. In this paper, we focus on two main families: the resonant and the scattered KBOs. The mean motion resonance can prevent the resonant KBOs from a close encounter with the Neptune. Thus most resonant KBOs are stable on their orbits. Here we mainly investigate the resonance width and strength of different resonant ratios with a high eccentricity. As for the scattered KBOs, their evolution is affected jointly by the resonance sticking and the resonance scattering. Here we are mainly concerned with the problem why there are a high number of KBOs in the scattered disc.
Keywords:
本文引用格式
蓝磊, 李俊峰.
LAN Lei, LI Junfeng.
柯伊伯带(Kuiper belt)全称艾吉沃斯-柯伊伯带(Edgeworth-Kuiper belt),是海王星(Neptune)轨道之外的一个小行星富集的盘状区域(轨道半长轴$a > 30.1$ AU)。对于柯伊伯带小行星(Kuiper belt objects,KBOs;又称Trans-Neptunian objects:TNOs)的研究,特别是统计学上的规律研究,对于理解太阳系的起源以及演化有着重要的意义,例如,可以反映太阳系在形成过程中大行星迁移的具体过程[1]。 由于受到太阳系最外围巨行星——海王星的强烈作用,柯伊伯带小行星的形成及演化与海王星存在紧密的联系。
柯伊伯带小行星大体可以分为以下5族:半人马座小行星(centaur)、共振小行星(resonant)、离散小行星(scattered)、孤立小行星(detached)和传统柯伊伯带小行星(classical)。它们之间的界线并不十分明确。一般认为一百万年内的平均近日点距离小于海王星半长轴的属于半人马座小行星。该类小行星的轨道常常穿越海王星轨道,轨道稳定性较差,一般只有几百万年到几千万年的寿命。离散小行星的近日点距离一般处在$30 \text{AU} < q < 37 \text{AU}$范围内($q$表示近日点距离,AU:AstronomicalUnit为一个天文单位)。由于近日点离海王星轨道较近,离散小行星的轨道受到海王星强烈摄动。在离散小行星的整个寿命中,至少会经历一次和海王星的近距离相遇。在与海王星近距离相遇之后,小行星的轨道根数常常发生较大改变。大部分小天体半长轴不断增大,远日点向太阳系外运动,甚至到达奥特星云(Oort cloud);小部分物体进入到海王星轨道以内,可能与其他行星发生碰撞。因此,离散小行星被认为是半人马座小行星、木星族小行星以及哈雷彗星的可能来源。离散小行星所处区域又被称为离散盘(scattereddisc)。离散小行星的轨道变化较为频繁,但令人惊讶的是,相比于更加稳定的柯伊伯带小行星(如传统柯伊伯带小行星),离散小行星的数量并不处于劣势。孤立小行星的近日点距离一般大于40 AU。由于离海王星相对较远,受海王星引力影响较小,动力学参数较为稳定,如同被孤立了一般。近日点距离在$37 \text{AU} < q < 40 \text{AU}$的区域通常被认为是离散小行星和孤立小行星共同存在的区域。传统柯伊伯带小行星未与海王星形成共振,它们又可以分为两类:一类为活跃的传统柯伊伯带小行星(hotclassical Trans-Neptunian objects),轨道半长轴一般处在$42 \text{AU} < a <47.5 \text{AU}$ ($q > 38 \text{AU}$)范围,最明显的特征是这些小行星的轨道倾角$i$大于$5^{\circ}$ (另有文献认为大于${10}^{\circ}$)。另一类为较稳定的传统柯伊伯带小行星(cold classical Trans-Neptunian objects),轨道半长轴一般处在$37 \text{AU} < a < 40 \text{AU}$ $(q > 37$ AU),与活跃小行星的区别在于这些小行星的轨道倾角$i$小于$5^\circ$ (另有文献认为小于$10^{\circ}$)。共振小行星分布范围较广,半长轴大小取决于所处具体共振域和轨道偏心率。
总而言之,孤立小行星和传统柯伊伯带小行星受海王星影响较小,半人马座小行星的寿命一般较短。共振小行星和离散小行星近日点距离海王星较近,这两族小行星受海王星影响较大且寿命较长(仿真模拟中,有些小天体的寿命甚至大于太阳系寿命45亿年)。本文重点讨论海王星共振效应对这两族小行星动力学特性的影响。
1 共振小行星
小行星与海王星共振是指小行星绕太阳公转周期与海王星公转周期成简单整数比。海王星引力对柯伊伯带小行星轨道有周期性作用。共振过程中,小行星公转轨道的拱线如同钟摆般振动。小行星与大行星形成共振需满足两个条件:一是两者的公转周期形成简单整数比,二是小行星共振角在共振中心附近周期振动[2-3]。某些情况下小行星的公转周期虽然与大行星公转周期形成相应整数比,但无法形成稳定共振角,就不属于共振状态。小行星与大行星形成共振可以避免与大行星近距离接触,因此轨道更稳定。小行星与大行星的轨道共振可分为外部共振(exterior resonance)和内部共振(interior resonance)。 外部共振的小行星公转周期大于海王星,轨道半长轴大于海王星。内部共振的小行星公转周期小于海王星,小行星轨道半长轴小于海王星。大部分柯伊伯带小行星半长轴都大于海王星,因此本文主要讨论外部共振。
影响小行星与行星共振稳定性的因素主要有行星质量和行星轨道偏心率。行星轨道偏心率越大,处在共振区域的轨道越趋于不稳定。行星质量越大,对小天体的引力作用越大。虽然质量较大的行星可以形成更宽的共振域,但如果共振域不稳定,扰动也更为明显。这与多个共振域发生重叠有关(resonance overlapping)。例如小行星带中的柯克伍德空隙,就是由木星内部$1:3$共振不稳定形成的。海王星与太阳的质量比相对较小,海王星公转偏心率也较小,所以柯伊伯带小行星与海王星可以形成较为稳定的共振。
与海王星形成$i:j$共振的小行星半长轴近似如下
其中$i$和$j$为正整数(外部共振$i > j$)。$a_{\text{N}}$为海王星的半长轴,约为30.1 AU。如$2:1$共振小行星半长轴约为$a_{\text{res}} = (2/1)^{{2}/{3}}a_{_\text{N} } = 1.587a_{\text{N}} =47.77$ AU。
已知的共振小行星与海王星形成多种共振比,包括了1:1 (海王星特洛伊族小行星), 5:4, 4:3, 11:8, 3:2, 18:11, 5:3, 12:7, 19:11, 7:4, 9:5,11:6, 2:1, 9:4, 16:7, 7:3, 12:5, 5:2, 8:3, 3:1, 4:1, 11:2, 5:1和27:4等等。甚至发现了如9:1这样大半长轴的共振小行星,同时也发现了诸如27:4这样复杂共振的小行星。发现小行星数量较多的共振比有1:1,3:2,2:1, 5:2, 5:3, 7:4等。
对于低偏心率小行星轨道(一般认为偏心率小于$0.3$),共振强度(strength of resonance)可用如下公式近似衡量
其中$S =\sin(i/2)$,$\alpha = (a/a_\text{N})^{-1}$,$f_d (\alpha )$和$f_i (\alpha)$为拉普拉斯因子[10-11]。当偏心率较小时,共振强度主要取决于$e^{j_4}$项,近似为$e^{i -j}$。所以,在小偏心率情况下,共振强度主要取决于表示共振比的两个整数的差值${\vert} i - j{\vert}$。当${\vert }i - j \vert=1$ 时,称这样的共振为一阶共振,如$2:1$和$3:2$共振。当$\vert i - j\vert=2$时,称这样的共振为二阶共振,如$3:1$和$5:3$共振。在小偏心率情况下,一般认为低阶共振比高阶共振强度更高。某些共振比下的共振区域,特别是共振比小于$4:3$的共振,常常发生共振重叠(resonance overlapping)。轨道相图中可以发现共振重叠区域往往覆盖着许多无序的区域,处在重叠区域的小天体轨道稳定性较低,往往不能长期处于单一共振域内。
过去对于柯伊伯带共振小行星的研究主要集中于小偏心率情况。观测表明大偏心率物体大量存在。对于具体共振域的确定,主要依靠庞卡莱截面计算共振的相图,测量相图中稳定域(stable island)的宽度来确定共振域(resonance width)。Malhotra [12]使用庞卡莱截面计算了$6:5$,$5:4$,$4:3$,$7:5$,$3:2$,$5:3$,$2:1$等共振比下的庞卡莱截面,并计算了相应的共振域,主要考虑偏心率小于$0.4$的情况。共振域反映了相应共振的影响范围。Wang等[13]所用的庞卡莱截面可使共振域计算拓展到大偏心率轨道,并确定全域下的共振域。在平面限制性三体模型下,Wang等[13]计算了$2:1$ 和$3:2$内部共振的宽度,并以此预测了第九大行星(未实际观测到)对于柯伊伯带小行星的影响。
$5:2$共振是三阶共振。根据过去的小偏心率理论,共振强度较弱。但观测结果表明海王星$5:2$共振域中存在着和$3:2$、$2:1$共振数量相当的小行星,见表1,且这些小行星多处于$e = 0.4$附近。可见传统理论并不适用于中偏心率及大偏心率问题。Malhotra等[14]在引入四个巨行星引力的力学模型下,对小行星$5:2$共振轨道进行了长时间的数值仿真,仿真发现使用限制性三体模型所确定的共振域与三维模型所确定的共振小行星生存区域高度一致,验证了文献[13]方法的准确性。每个共振比下往往有多个共振子域,例如$5:2$共振存在着3个共振子域,分别为Zone I-1,Zone I-2 和Zone II (子域划分在文献[14]中有详细介绍)。该文献还将$5:2$外部共振的共振域范围与$2:1$和$3:2$共振对比。$5:2$共振域大小与$2:1$,$3:2$共振并没有明显区别,可以解释为何$5:2$共振与两个一阶共振拥有数量相当的共振小行星,见表1。在大偏心率情况下,过去的小偏心率理论并不适用。共振域宽度不再取决于$\vert i - j|$的大小。实际上更取决于$j$的大小。下面一节中对此将有进一步的介绍。
2 离散小行星
若使近日点距离$q$保持相对恒定,小行星公转半长轴变大,偏心率也随之变大,同时公转周期也变大。半长轴变小,偏心率也减小,公转周期变小。据推算,目前离散盘小天体大约只占在太阳系寿命中被离散的小天体总数的$1\text{\%}$。最初该族小行星可能形成于海王星目前所处位置附近($a \sim 30.1\;\text{AU}$)。在这颗巨行星向外迁移过程中,小行星逐渐被离散。在离散小行星的整个寿命中,至少会经历一次和海王星近距离相遇。公转半长轴大的小行星与海王星近距离相遇的周期较大。与海王星近距离相遇时,由于行星的引力作用,一部分离散小行星半长轴不断增大,小行星不断向深空移动。
在离散的过程中,共振黏滞(resonance sticking)效应尤为明显。共振黏滞是指离散小行星在被离散过程中半长轴不断增大,进入到当地共振域,受当地共振影响,一定时间内停留在共振域附近的现象,又称暂时性共振捕获(temporary resonance capture)。小行星被捕获的时间可长可短,长可超过一千万年,甚至成为长期共振的小行星。离散小行星远地点在向外移动过程中,经历的共振黏滞呈现法雷序列。例如:小行星经历$9:1$共振粘滞之后,在经历$10:1$共振黏滞之前,往往会经历$(9+10):(1+1)=19:2$共振黏滞,接下去可能会经历$(19+10):(2+1)=29:3$共振黏滞,以此类推。共振黏滞的机理较为复杂,目前未有定论,过去的研究主要集中于半解析分析或数值仿真[20-31]。
离散小行星的远地点在远离太阳的过程中,交替经历着离散和共振黏滞。根据Lykawka的模拟结果,在离散小行星的整个寿命中,约有38{\%}的时间处在共振黏滞中。模拟发现共振黏滞作用在250 AU范围内较为明显。一些小行星可能在一个共振域周围长时间运动,共振角稳定的时间超过了所设定的积分判别时间,这也给准确区分离散小行星和共振小行星造成一定难度。
Lykawka以共振黏滞的捕获机率和捕获总时间来定义黏滞强度,数值计算发现,在离散盘中,共振黏滞最为强大的依次为$n:1$,$n:2$和$n:3$共振域,并以此类推。即:共振比(表示为$i:j$)的$j$越小,该共振的黏滞作用越强,这与上一节共振小行星中的结论一致。此外,共振黏滞的强度随着$j$增大而快速衰减。因此,只有$n:1$,$n:2$和$n:3$三个$j$值较小的共振黏滞效应较为明显。大偏心率情况下共振黏滞强度不再取决于$|i - j|$,而是取决于$j$。
仿真发现,如果一颗小行星在单一共振比里经历了较长时间的共振黏滞,近日点距离$q$ 有可能被抬升,以致于变成共振小行星或孤立小行星,该现象被称为$q-$提升效应。对于$n:1$,$n:2$和$n:3$共振,$q-$提升效应主要发生在半长轴小于$250 \text{AU}$,$200 \text{AU}$和$170 \text{AU}$的范围之内。在这些范围之外,$q-$提升效应不明显。
==图1展示了数个共振比下的共振域宽度。图中接近横向的灰色实线根据天王星公转轨道画出,灰色实线所代表的轨道与海王星轨道交叉($q = 19.22$ AU)。当小行星轨道与天王星轨道交叉时,即小行星轨道近日点进入天王星轨道以内,常常受到天王星引力强烈作用,轨道变得不稳定。所以,一般考虑图中这条实线以下的区域。该区域中的小行星轨道都处在天王星轨道之外。在全模型中模拟得到,一般在$q > 26$ AU的情况下容易形成稳定共振。
图1
如图1黑色实线所示,$n:1$共振的最大共振域出现在线$a(1 - e) =1$附近(公式中的"1"为归一化的数值,代表海王星半长轴长度,约30.1 AU),即小行星的近日点距离与海王星轨道半径相近时,$n:1$共振的共振域宽度最大。如图1中黑色点划线所示,$n:2$ 共振的最大共振域出现在$a(1- e) = 1.1$附近,近日点距离接近33.1 AU。如图1中灰色点划线所示,$n:3$共振的最大共振域出现在$a(1 - e) =1.15$附近,近日点距离接近34.6 AU。 这些共振比下的最大共振域都发生在$30 \text{AU}$到$37\text{ AU}$以内,即离散盘以内。一方面,离散盘中的小行星轨道近日点距离接近于海王星轨道,常常与海王星近距离交会,受海王星的离散作用明显。另一方面,主要共振的最大共振域宽度又出现在离散盘,这就不难理解如此多的小行星存在于离散盘中。这些离散小行星在演化过程中不断交替经历着离散和共振黏滞。
3 总结
柯伊伯带小行星的研究对于探索太阳系的起源和演化有着重要意义。共振小行星和离散小行星近日点距离海王星轨道较近,受海王星引力影响较为明显。共振可使小行星避免与海王星近距离交会,轨道保持相对稳定。目前发现小行星数目较多的共振比有$1:1$,$3:2$,$2:1$,$5:2$共振等。对于共振强度的估计,传统的理论模型只适用于小偏心率情况,在大偏心率情况下,共振强度主要由数值方法来确定。Wang和Malhotra 使用的庞卡莱截面能够较为精确地确定共振域的宽度。研究发现高阶共振在大偏心率情况下也有与低阶共振相比拟的共振宽度。离散小行星的演化过程中交替经历着离散和共振粘滞,$n:1$,$n:2$和$n:3$共振的黏滞最为明显,最大共振宽度处于$30 \text{AU} < q < 37 \text{AU}$范围,正好处于离散盘之内,共振的黏滞作用使得小天体在离散盘内富集。
参考文献
Tracking Neptune's migration history through high-perihelion resonant trans-Neptunian objects
Origin of scattered disk resonant TNOs: evidence for an ancient excited Kuiper belt of 50 AU radius
The origin of Pluto's orbit: implications for the solar system beyond Neptune
Planetary migration and Plutino orbital inclinations
Planetary migration in a planetesimal disk: why did Neptune stop at 30 AU
The origin of the Kuiper Belt high-inclination population
The Kuiper belt extends from the orbit of Neptune at 30 au to an abrupt outer edge about 50 au from the Sun. Beyond the edge is a sparse population of objects with large orbital eccentricities. Neptune shapes the dynamics of most Kuiper belt objects, but the recently discovered planet 2003 VB12 (Sedna) has an eccentric orbit with a perihelion distance of 70 au, far beyond Neptune's gravitational influence. Although influences from passing stars could have created the Kuiper belt's outer edge and could have scattered objects into large, eccentric orbits, no model currently explains the properties of Sedna. Here we show that a passing star probably scattered Sedna from the Kuiper belt into its observed orbit. The likelihood that a planet at 60-80 au can be scattered into Sedna's orbit is about 50 per cent; this estimate depends critically on the geometry of the fly-by. Even more interesting is the approximately 10 per cent chance that Sedna was captured from the outer disk of the passing star. Most captures have very high inclination orbits; detection of such objects would confirm the presence of extrasolar planets in our own Solar System.
The outer solar system origins survey: I. Design and first-quarter discoveries
The resonant trans-Neptunian populations
The $5:1$ Neptune resonance as probed by CFEPS: dynamics and population
The occurrence of high-order resonances and Kozai mechanism in the scattered disk
The phase space structure near neptune resonances in the Kuiper belt
Mean motion resonances at high eccentricities: the $2:1$ and the $3:2$ interior resonances
Neptune's $5:2$ Resonance in the Kuiper belt
We present numerical simulations of giant planet migration in our solar system and examine how the speed of planetary migration affects inclinations in the resulting population of small bodies (test particles) scattered outward and subsequently captured into Neptune's 3:2 mean motion resonance (the Plutinos) as well as the hot classical Kuiper belt population. We do not find a consistent relationship between the degree of test particle inclination excitation and e-folding planet migration timescales in the range 5 - 50 Myr. Our results present a counter-example to Nesvorný (2015)'s finding that the Plutino and hot classical inclinations showed a marked increase with increasing e-folding timescales for Neptune's migration. We argue that these differing results are likely due to differing secular architectures of the giant planets during and after migration. Small changes in the planets' initial conditions and differences in the numerical implementation of planet migration can result in different amplitudes of the planets' inclination secular modes, and this can lead to different final inclination distributions for test particles in the simulations. We conclude that the observed large inclination dispersion of Kuiper belt objects does not require Neptune's migration to be slow; planetary migration with e-folding timescales of 5, 10, 30, and 50 Myr can all yield inclination dispersions similar to the observed Plutino and hot classical populations, with no correlation between the degree of inclination excitation and migration speed.
The scattered disk as the source of the Jupiter family comets
OSSOS III——Resonant trans-Neptunian populations: constraints from the first quarter of the Outer Solar System Origins Survey
Dynamical classification of trans-Neptunian objects: probing their origin, evolution, and interrelation
Exploring the $7:4$ mean motion resonance——II: scattering evolutionary paths and resonance sticking
Resonance sticking in the scattered disk
Asymmetric librations in exterior resonances
The resonant structure of the Kuiper belt and the dynamics of the first five trans-Neptunian objects
Chaotic diffusion and the origin of comets from the 2/3 resonance in the Kuiper belt
The phase space structure near Neptune resonances in the Kuiper belt
Mean motion resonances in the trans- Neptunian region: I:the $2:3$ resonance with Neptune
Mean motion resonances in the trans-Neptunian region: Part II: the $1: 2$, $3: 4$, and weaker resonances
Frequency map and global dynamics in the Solar System I: short period dynamics of massless particles
On the Plutinos and Twotinos of the Kuiper belt
We present numerical simulations of giant planet migration in our solar system and examine how the speed of planetary migration affects inclinations in the resulting population of small bodies (test particles) scattered outward and subsequently captured into Neptune's 3:2 mean motion resonance (the Plutinos) as well as the hot classical Kuiper belt population. We do not find a consistent relationship between the degree of test particle inclination excitation and e-folding planet migration timescales in the range 5 - 50 Myr. Our results present a counter-example to Nesvorný (2015)'s finding that the Plutino and hot classical inclinations showed a marked increase with increasing e-folding timescales for Neptune's migration. We argue that these differing results are likely due to differing secular architectures of the giant planets during and after migration. Small changes in the planets' initial conditions and differences in the numerical implementation of planet migration can result in different amplitudes of the planets' inclination secular modes, and this can lead to different final inclination distributions for test particles in the simulations. We conclude that the observed large inclination dispersion of Kuiper belt objects does not require Neptune's migration to be slow; planetary migration with e-folding timescales of 5, 10, 30, and 50 Myr can all yield inclination dispersions similar to the observed Plutino and hot classical populations, with no correlation between the degree of inclination excitation and migration speed.
Comparative study of the $2:3$ and $3:4$ resonant motion with Neptune: an application of symplectic mappings and low frequency analysis
The dynamics of the $1:2$ resonant motion with Neptune in the 3D elliptic restricted three-body problem
Resonances in the asteroid and trans-Neptunian belts: a brief review
/
〈 |
|
〉 |
