结构优化技术在高速飞行器上的应用与面临的挑战 1)
*
†
**
APPLICATIONS AND CHALLENGES OF STRUCTURAL OPTIMIZATION IN HIGH-SPEED AEROCRAFT 1)
*
†
**
通讯作者: 2) 关成启,研究员,主要研究方向为飞航武器系统总体设计。E-mail:guanchengq@126.com3) 张卫红,教授,主要研究方向为结构优化设计理论、方法及其应用。E-mail:zhangwh@nwpu.edu.cn
收稿日期: 2018-11-12 网络出版日期: 2019-08-27
基金资助: |
|
Received: 2018-11-12 Online: 2019-08-27
作者简介 About authors
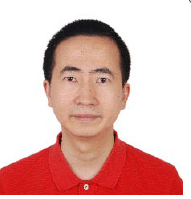
全栋梁,浙江杭州人,研究员2005年西北工业大学获博士学位长期从事高速飞行器结构与热防护技术研究,在国内外核心期刊发表文章20多篇,发明专利5项研究方向为先进飞行器结构、防热与强度的方法与应用。
高速飞行器是航空航天领域发展的重要方向,具有重大战略意义和重要经济社会价值。首先,对结构优化技术及其在飞行器零部件和全机结构概念设计中的应用进行综述;然后,根据典型使用环境和设计要求,总结高速飞行器结构设计中存在的主要问题和应用需求,着重介绍近年来在高速飞行器零部件和全机结构概念设计中应用结构优化技术的典型案例;最后,提出近期亟待突破或完善的结构优化技术。结构优化技术与设计经验相结合带来设计理念的变革,将成为先进飞行器设计的必备工具和标准流程。面向重大需求的结构优化理论研究与工程实践对提升我国航空航天核心竞争力具有重要价值。
关键词:
With its great national strategic importance and socio-economic benefits, the high-speed vehicle always plays a specific role in the field of aerospace. In this paper, the structural optimization technique and its application in the aircraft design are reviewed firstly. Then, the specific applications of structural optimization techniques in the high-speed vehicle design are then discussed in the context of the typical service environment and the design requirement. We show typical cases subsequently with applications in the concept design of the high-speed vehicle and in the innovative and improved design of their parts over the last years. In view of the solid theoretical foundations as well as the numerous successful engineering practices of the structural optimization, it not only provides an effective tool, but also brings about revolutionary changes for the design of high-speed vehicle structures. It is anticipated that the structural optimization supplemented by the empirical design will surely be a standard procedure for the aircraft design, and the practice-oriented research will undoubtedly enhance the core competitiveness of the aerospace industry in China
Keywords:
本文引用格式
全栋梁, 时光辉, 关成启, 王健, 罗俊航, 宋锋, 王博, 高彤, 朱继宏, 张卫红.
QUAN Dongliang, SHI Guanghui, GUAN Chengqi, WANG Jian1, LUO Junhang, SONG Feng, WANG Bo, GAO Tong, ZHU Jihong, ZHANG Weihong.
引言
高速飞行器是航空、航天领域发展的重要方向,具有重大战略意义和重要经济社会价值。未来几年到几十年,武器装备、飞机、空天往返飞行器等航空航天装备面临着全面更新换代,而高速化是其重要趋势,具有代表性的方案包括中国航天科工集团空天往返飞行器(图1)、SR-72高超音速概念飞机等[1]。SR-72能够以超过5 马赫的速度在临近空间长时间飞行,甚至穿梭于大气层与外太空轨道之间。高速飞行器在整个使用过程中必须经受复杂严酷的载荷环境。一方面,在大气层内高速飞行过程中,高速飞行器因气动加热表面从常温逐渐升到极端高温,例如6马赫飞行的高速飞行器前缘驻点温度超过1500K,局部短时温度梯度高达700K/cm极端环境下短时温升速率达到50K/s;另一方面,除了高温和气动载荷引起的结构变形,各种原因引起的振动是高速飞行器设计必须考虑的另一关键因素,例如局部内外流耦合部位振动总均方根量级超过20$g$,局部噪声环境超过165dB。综合而言,与传统飞行器相比,高速飞行器的使用环境异常严酷。
图1
不同于传统的弹道型飞行器,高速飞行器必须在极高的飞行速度下满足远射程、长航时、高机动等性能要求,对飞行器结构系统轻量化设计提出了严峻挑战,必须进一步降低结构质量系数。结构质量系数为飞行器结构系统质量相对起飞质量的比值,是衡量飞行器结构轻量化的指标。目前,亚声速导弹的结构质量系数约为22%~25%,超声速导弹的结构质量系数约为25%~33%,飞行速度越高结构轻量化设计的难度越大,未来飞行器增加热防护及热控系统后结构质量系数要降低到20%以下才能使系统方案具备优势。与传统飞行器相比,高速飞行器因使用环境温度和机动性要求更高,载荷条件更为严酷。如何在满足苛刻使用性能要求的前提下降低结构质量系数,关系到高速飞行器性能指标的实现与全面提升。
本文着重介绍工程应用探索案例,并指出结构优化技术在高速飞行器设计中所面临的严峻挑战。
1 结构优化技术及其在飞行器设计中的应用
1.1 结构优化设计
结构优化设计是在满足各种规范或给定性能要求的条件下使结构的某种广义性能指标(如质量、造价、刚度或频率等)最优,或者说在所有可用的方案中,按照某种标准找出最优的方案[4]。
结构优化从设计变量上分类,可分为尺寸优化、形状优化、拓扑优化三大类。对于研制类项目,分别对应结构系统研制的详细设计阶段、基本设计阶段、概念设计阶段(如图2所示)。研究和工程应用表明,三类优化设计中,拓扑优化由于不受初始构型的限制,可以得到比尺寸优化和形状优化更显著的减重和性能改进效果,然而,随着新的优化理论的不断出现,三者的界限逐渐变得模糊。
图2
1.2 结构优化技术在飞行器设计中的应用
图3
1.2.1零件设计
零件作为结构中最小承载单元,载荷形式相对简单、边界相对清晰,非常适合采用结构优化手段实现减重和结构性能提升。
涡轮发动机接头结构拓扑优化,考虑传统工艺限制,在保证结构强度和刚度指标条件下,实现结构减重25%;采用增材制造工艺,最大位移降低50%的同时实现结构减重66%,效果显著[52]。Fairchild Dornier728飞机舱门支撑臂拓扑优化,考虑结构的可制造性、在保证结构刚度基础上减重20%,开发周期从原来的三个月缩短到三个星期[53]。除上述航空飞行器零件设计应用外,国防科技大学采用拓扑优化对"天拓3"微型卫星支座进行了优化设计;优化后结构除轴向频率略有降低外,最大应力、一阶失稳载荷因子、基频均得到改善,与原始设计模型相比结构减重17.93%,相比以往"经验仿真试验"的迭代手段,有效降低了设计周期和试验成本[46]。
结构优化特别是拓扑优化技术在零件设计中的应用主要集中在接头、铰链、支架等集中传载结构上,应用效果上能够在保持甚至提升结构性能的基础上,大幅降低结构质量、提高设计效率。
1.2.2部件设计
国内某大型飞机发动机吊挂采用拓扑优化设计获得了类桁架的传力结构布局形式[47],Samtech公司(已现被西门子公司收购)为空客A350开展的发动机吊挂设计工作[48]获得了类似的结构布局形式,异曲同工的设计结果反映出此类集中传力部件采用类桁架结构传载效率较高。A350后机身结构优化,采用拓扑优化获得的布局方案抛弃了传统的框式结构,获得一种包含剪力墙和空间压杆的混合式新结构,与A330相比在结构应力水平显著降低的同时整体减重达20%[54]。空客A33机翼承力盒结构优化设计获得了明显的减重效果,以此为基础开发的专用优化流程已推广到大量其他机型、不同材料(金属和复合材料)以及其他部件(机身、机翼、尾翼、发动机挂架)的设计改进中[49]。在空客A400军用运输机整个后机身传力路线和机身框桁架结构设计中的应用方式是首先开展多轮优化获得机身传力路线,在此基础上针对重点部位开展局部优化设计以及参数优化,能够通过降低迭代次数降低开发成本[55]。对波音777全尺度机翼结构进行的拓扑优化,采用了超大规模并行计算,获得了类仿生结构机翼设计方案,预计可实现单个机翼减重2%~5%,对应结构质量为200kg~500kg[56]。
部件结构优化设计目前的应用类型相对宽泛,包括挂架、舱段、机身框架、机翼等大型部件。从零件到部件,结构优化设计问题复杂程度显著提高,结构减重百分比有所减小,但减重的绝对数值大幅增加效果更为显著。
1.2.3全机概念设计
结构优化设计能够在结构设计的各个阶段发挥作用,其中拓扑优化在结构构型或布局方案未确定情况下,越早介入越能够获得更大收益。伦敦玛丽女王大学学者和Altair工程师将拓扑优化应用于翼身融合的民用载人飞机和无人军用飞机的整机结构概念设计,获得了整机的传力路线框架布局,进一步结合尺寸和形状优化形成了整机结构设计方案,验证了这一技术手段和思路对于全新飞行器结构设计的有效性[57]。华中科技大学学者针对飞行器承载构型开展拓扑优化研究,在保证飞行器结构指标满足约束的情况下,通过理论分析,飞行器机身质量由3.054t降至1.947t,一阶固有频率由293Hz提高到515Hz[58]。将拓扑优化用于全机结构概念设计,结合尺寸和形状优化进行详细设计,为飞行器结构设计提供了新的技术手段。
2 结构优化技术在高速飞行器设计中的应用
2.1 高速飞行器结构设计需求
高速飞行器必须满足严酷使用条件下苛刻的技术指标,这将带来一系列的工程技术难题,包括:局部超高结构温度、局部大温度梯度、急速的升温和降温速率、更低的结构质量系数、更高的局部动响应要求等。相应地,对面向工程应用的结构优化设计提出了更强烈和迫切的需求,主要包括:
(1)复杂结构局部超高温下热扩散问题:对于复杂结构局部超高结构温度,除了在耐高温材料上攻关外,如何通过合理的结构设计实现热量的迅速扩散、降低局部结构温度,至关重要。
(2)大温度梯度下结构设计问题:局部大温度梯度带来的是严重的热应力集中问题,同时热应力不同于机械力载荷,加强结构有时会进一步恶化应力状态、导致结构局部破坏。
(3) 大型整体结构一体化问题:大型承载结构一体化设计与制造、减少工艺分离面与连接增重,对于降低结构系统减重至关重要,甚至可以说大型承载结构的轻量化水平决定了整个飞行器的轻量化水平。
(4) 设备布局优化问题:不同设备对于外部的环境要求不同,如何布置设备能使得设备环境最佳;同时,对于具有一定承载能力的设备,将其纳入到结构传力路径当中,能够最大限度提升一体化水平,降低结构质量系数。
(5) 结构极致轻量化设计问题:对于中小尺度非大承载部件,在满足功能需求的基础上,采用点阵、蜂窝、夹层等轻量化结构,结合优化设计方法、新型增材制造工艺,实现结构极致轻量化是未来结构设计的重要方向。
(6) 复合材料结构设计问题:高速飞行器更多地使用陶瓷基和树脂基复合材料已成为一种必然趋势,由于其不同于金属材料的力学性能,必须针对性发展复合材料结构优化设计技术才能最大限度发挥其优势。
(7) 窗口局部保形设计问题:高速飞行器必须具备高机动性和信息探测/感知能力,因此进气道、透光透波窗口等结构在复杂的多场耦合载荷工况下的局部变形控制,直接关系到上述性能的优劣。
(8) 动载荷下结构设计问题:高速飞行器工作在高度复杂的热声振耦合环境下,一方面必须通过优化设计有效降低结构关键位置动响应、为机载设备提供良好环境,另一方面动载荷引起的疲劳问题是可重复使用高速飞行器设计必须考虑的关键问题。
(9) 不确定性(可靠性)结构优化设计问题:针对未来高速飞行器结构可重复使用结构、以及使用环境、原材料、加工、制造等环节的不确定性因素,开展结构优化设计至关重要。
(10) 面向加工制造的结构优化问题:现有商业软件算法大多基于变密度法,获得的结果为基于像素点的隐式拓扑描述,获得拓扑结果后要进行几何模型重构获得结构化模型。这一思路目前基本能够满足工程需求,但也存在明显不足。一方面,模型重构过程不可避免会丢失几何信息,使最优结构在一定程度上打了折扣;另一方面,重构效率低且对技术人员的知识积累依赖程度较高,往往需要在重构与数值校核之间迭代多次。因此,建立基于显式几何描述、能够自动生成结构化模型、考虑制造工艺约束的新型拓扑优化算法,是未来结构优化设计的重要发展方向。
以上从零部件、结构总体以及特定工程角度列出了高速飞行器结构设计技术的重点需求方向,相信还会出现更多的应用需求。可以确定,结构优化设计技术正在成为高速飞行器结构设计的必备技术和环节。
2.2 结构优化技术在高速飞行器设计中的应用
目前,国内外采用结构优化技术开展高速飞行器结构设计的公开报道极少。近年来,围绕工程研制需求,北京空天技术研究所与西北工业大学和大连理工大学合作,开展了结构优化理论方法及其在高速飞行器结构设计中的应用研究,包括零件(发动机安装接头、舵轴等)、部件(舵面等)以及全机结构概念设计。
2.2.1 零件设计
发动机安装接头用于传递发动机推力,布置在发动机推力传递截面、发动机两侧左右各一个成对使用。该安装接头为集中承载部件,除了要保证其自身强度、刚度以及耐温性要求以外,功能上要实现发动机与机身结构的有效连接及载荷的可靠传递。根据对接头结构形式的分析,采用拓扑优化结合尺寸优化方法开展结构优化设计(图4)。重点考虑结构承载性能和轻量化水平,同时兼顾安装可操作性;构件以增材制造工艺加工,故设计中未特别考虑工艺限制。优化后结构刚度指标略有提升,局部最大应力略增、但仍在安全范围内;与面向传统机加工艺的原设计方案相比,减重超过18%。
舵轴初始设计采用分段机加、焊接连接工艺(图5)。根据对舵轴结构形式的分析,依据整体结构拓扑优化结果,进一步应用尺寸优化减重设计与增材制造工艺加工,满足了所有性能要求,减重达8.7%。
图4
图5
零件结构优化设计在工程关键技术攻关阶段实现了改进或原创设计,在保证承载性能的基础上,取得了显著的减重效果。结合金属增材制造工艺,极大缩短了零件设计、制造与验证周期,初步实现了面向性能的结构设计。与以往面向工艺的结构设计相比,性能较少向工艺妥协,设计自由度更大,减重效果更好。
2.2.2 部件设计
图6
舵面作为独立部件,在高温与压力联合作用下,必须满足刚度、强度和转动惯量等指标。首先采用拓扑优化获取主传力路径,结合设计人员经验提取结构特征,通过尺寸参数优化对重构模型进行详细设计,使结构减重约10%。
结构设计人员在方案论证阶段可以利用部件优化设计方法对结构进行改进。应用案例表明结构优化技术与工程经验相结合,能得到符合功能需求、性能优异且具备工程实现条件的结构。
2.2.3 全机概念设计
在概念设计阶段应用结构优化技术开展结构概念方案构建是一项具有挑战性的工作。基于工程前期研究基础,探索了一种基于拓扑优化的高速飞行器全机结构概念设计方法(图7)。首先,根据载荷和设计目标估计出初步的最大变形、质心位置、最小一阶频率设计要求,通过拓扑优化快速获得结构质量指标的可实现下限,从而确定一组可用于进一步结构设计的系统性指标;然后,在拓扑优化设计结果基础上,建立参数优化模型(考虑功能需求,如预留设备空间、增加操作口盖等);最后,根据上述结构系统性指标建立优化设计约束,以结构系统整体刚度最大作为设计目标,开展尺寸优化设计获得全机参数化模型。在结构概念设计阶段经过以上两轮结构优化,获得的飞行器全机结构概念设计方案可作为后期结构详细设计阶段的重要参考。
图7
2.3 亟需突破的结构优化技术
结构优化方法作为创新设计手段,在高速飞行器结构设计应用探索中已经展现出巨大的能力与潜力。然而,目前的应用仍以冷结构和中低温热结构为主,结构性能主要考虑刚度、强度和固有频率;材料以金属为主,局部使用复合材料;拓扑优化设计结果的解读和特征提取仍依赖于设计人员的手动操作,工艺约束考虑较少;全机概念设计中受限于计算能力,模型规模较小,设计结果粗糙。
为满足高速飞行器极端载荷环境和高性能指标要求,结构优化技术近期亟待突破或完善以下技术:
(1) 建立局部超高温热扩散结构设计技术;
(2) 完善大梯度高温热结构拓扑优化技术;
(3) 完善动载荷下的结构拓扑优化技术,实现局部动响应特性改善;
(4) 发展全新的拓扑优化方法,避免密度法拓扑优化结果特征提取带来的性能损失;
(5) 发展面向增材制造的拓扑优化技术,建立增材制造结构件性能评估和质量控制标准;
(6) 发展陶瓷基复合材料结构优化设计技术;
(7) 进一步针对未来复杂可重复使用结构优化问题,完善不确定结构优化设计方法;
(8) 基于并行计算发展超大规模结构高性能分析与优化设计平台,实现大型部件和全机的精细概念设计。
3 结束语
结构优化技术在国内外航空航天领域已经得到大量应用,尤其是拓扑优化技术在提高结构性能、降低结构质量中已发挥出显著作用,被行业普遍接受。拓扑优化技术的应用已从肋板、支架、挂架等零件推广到机身、部段甚至整机的优化设计。高速飞行器由于其极端使用环境和严苛性能要求,对结构优化技术具有迫切需求。结构优化技术在零部件以及全机概念设计中具有极大潜力,但仍需要开展更为深入的理论方法和应用基础研究。
大量理论方法研究和工程应用实践表明结构优化技术不但为飞行器结构研制提供了有效的设计工具,更重要的是带来设计理念的变革。
(1) 结构优化技术正逐步成为先进飞行器设计过程必备工具和标准流程;
(2) 结构优化设计与传统经验相结合在高性能轻量化应用中能发挥出更大的作用;
(3) 结构优化设计在飞行器研制的详细设计阶段、基本设计阶段、概念设计阶段均能发挥作用;
(4) 拓扑优化技术与增材制造工艺的有机融合,能够实现从无到有的设计,最大限度发挥结构优化的作用,将成为未来结构设计与制造的组合技术,实现真正的面向性能的结构设计。
参考文献
Topology optimization in aircraft and aerospace structures design
Optimal shape design as a material distribution problem
Recent development in structural design and optimization
With the fast development of computational mechanics and thecapacity as well as the speed of modern computers, simulationbased structural optimization has become an indispensable tool inthe design process of competitive products. This paper presents abrief description of the current status of structural optimizationby reviewing some significant progress made in the last decades.Potential research topics are also discussed. The entireliteratures of the field are not covered due to the limitation ofthe length of paper. The scope of this review is limited andclosely related to the authors' own research interests.
Generating optimal topologies in structural design using a homogenization method
A 99 line topology optimization code written in Matlab
<a name="Abs1"></a>The paper presents a compact Matlab implementation of a topology optimization code for compliance minimization of statically loaded structures. The total number of Matlab input lines is 99 including optimizer and Finite Element subroutine. The 99 lines are divided into 36 lines for the main program, 12 lines for the Optimality Criteria based optimizer, 16 lines for a mesh-independency filter and 35 lines for the finite element code. In fact, excluding comment lines and lines associated with output and finite element analysis, it is shown that only 49 Matlab input lines are required for solving a well-posed topology optimization problem. By adding three additional lines, the program can solve problems with multiple load cases. The code is intended for educational purposes. The complete Matlab code is given in the Appendix and can be down-loaded from the web-site http://www.topopt.dtu.dk.
Efficient topology optimization in MATLAB using 88 lines of code
The paper presents an efficient 88 line MATLAB code for topology optimization. It has been developed using the 99 line code presented by Sigmund (Struct Multidisc Optim 21(2):120-127, 2001) as a starting point. The original code has been extended by a density filter, and a considerable improvement in efficiency has been achieved, mainly by preallocating arrays and vectorizing loops. A speed improvement with a factor of 100 is obtained for a benchmark example with 7,500 elements. Moreover, the length of the code has been reduced to a mere 88 lines. These improvements have been accomplished without sacrificing the readability of the code. The 88 line code can therefore be considered as a valuable successor to the 99 line code, providing a practical instrument that may help to ease the learning curve for those entering the field of topology optimization. The paper also discusses simple extensions of the basic code to include recent PDE-based and black-and-white projection filtering methods. The complete 88 line code is included as an appendix and can be downloaded from the web site www.topopt.dtu.dk.
A simple evolutionary procedure for structural optimization
连续体结构拓扑优化设计的ICM方法
ICM method for topology optimization design of continuum structures
基于ICM方法三维连续体结构拓扑优化
ICM method for topology optimization design of continuum structures
A level set method for structural topology optimization
Structural optimization using sensitivity analysis and a level-set method
"Color" level sets: a multi-phase method for structural topology optimization with multiple materials
Phase field: a variational method for structural topology optimization
Doing topology optimization explicitly and geometrically: a new moving morphable components based framework
Temperature-constrained topology optimization of thermo-mechanical coupled problems
Integrated layout design of multi-omponent system
Feature-driven topology optimization method with signed distance function
A comprehensive study of feature definitions with solids and voids for topology optimization
Topology optimization with closed B-splines and Boolean operations
Explicit three dimensional topology optimization via Moving Morphable Void (MMV) approach
Topological design of freely vibrating continuum structures for maximum values of simple and multiple eigenfrequencies and frequency gaps
<a name="Abs1"></a>A frequent goal of the design of vibrating structures is to avoid resonance of the structure in a given interval for external excitation frequencies. This can be achieved by, e.g., maximizing the fundamental eigenfrequency, an eigenfrequency of higher order, or the gap between two consecutive eigenfrequencies of given order. This problem is often complicated by the fact that the eigenfrequencies in question may be multiple, and this is particularly the case in topology optimization. In the present paper, different approaches are considered and discussed for topology optimization involving simple and multiple eigenfrequencies of linearly elastic structures without damping. The mathematical formulations of these topology optimization problems and several illustrative results are presented.
A comparative study of dynamic analysis methods for structural topology optimization under harmonic force excitations
Topology optimization of continuum structures with local stress constraints
A new approach for the solution of singular optima in truss topology optimization with stress and local buckling constraints
<a name="Abs1"></a>The present paper investigates problems of truss topology optimization under local buckling constraints. A new approach for the solution of singular problems caused by stress and local buckling constraints is proposed. At first, a second order smooth-extended technique is used to make the disjoint feasible domains connect, then the so-called ε-relaxed method is applied to eliminate the singular optima from problem formulation. By means of this approach, the singular optimum of the original problem caused by stress and local buckling constraints can be searched approximately by employing the algorithms developed for sizing optimization problems with high accuracy. Therefore, the numerical problem resulting from stress and local buckling constraints can be solved in an elegant way. The applications of the proposed approach and its effectiveness are illustrated with several numerical examples.
Topology optimization with design-dependent loads
Topological optimization of continuum structures with design-dependent surface loading-part I: new computational approach for 2D problems
Topology optimization of heat conduction problem involving design-dependent heat load effect
<h2 class="secHeading" id="section_abstract">Abstract</h2><p id="">Topology optimization of steady heat conduction problem under both design-independent and design-dependent heat loads is studied by means of a modified bidirectional evolutionary structural optimization (BESO) method. Two types of problems are distinguished by their physical meanings and particularly design-dependent load effect is highlighted in the following two points. At the stage of sensitivity analysis, both the heat conductivity matrix and the design-dependent heat generation load associated with the void element are penalized in the same manner. The rationality is illustrated based on numerical tests. Furthermore, as the sensitivity of the objective function changes its sign during the iteration, a modified BESO procedure is presented to deal with the non-monotonicity of the objective function defined by the heat potential capacity. Detailed steps of the BESO procedure are presented for the element removal and growth while the inequality volume constraint is imposed. To conclude the work, numerical results and the element sensitivity obtained are discussed to show the effect of design-dependent load.</p>
A review about the engineering design of optimal heat transfer systems using topology optimization
Topology optimization of conductive heat transfer devices: an experimental investigation
Topology optimisations for integrated thermal protection systems considering thermo-mechanical constraints
Topology optimization for minimizing the maximum temperature of transient heat conduction structure
Topology optimization involving thermo-elastic stress loads
Structural topology optimization of thermo-elastic problems is investigated in this paper. The key issues about the penalty models of the element stiffness and thermal stress load of the finite element model are highlighted. The penalization of thermal stress coefficient (TSC) measured by the product between thermal expansion coefficient and Young’s modulus is proposed for the first time to characterize the dependence of the thermal stress load upon the design variables defined by element pseudo-densities. In such a way, the element stiffness and the thermal stress load can be penalized independently in terms of element pseudo-density. This formulation demonstrates especially its capability of solving problems with multiphase materials. Besides, the comparison study shows that the interpolation model RAMP is more advantageous than the SIMP in our case. Furthermore, sensitivity analysis of the structural mean compliance is developed in the case of steady-state heat conduction. Numerical examples of two-phase and three-phase materials are presented.
Multi-scale concurrent material and structural design under mechanical and thermal loads
Development of Pareto topology optimization considering thermal loads
智能复合材料的应用与力学研究进展
Advances in application and mechanics of intelligent composite materials
Integrated topology optimization with embedded movable holes based on combined description by material density and level sets
Homogenization-based topology optimization for high-resolution manufacturable microstructures
Optimal truss and frame design from projected homogenization-based topology optimization
Revisiting density-based topology optimization for fluid-structure-interaction problems
A density-based topology optimization methodology for thermoelectric energy conversion problems
A survey of structural and multidisciplinary continuum topology optimization: post 2000
Fail-safe topology optimization
Multi-objective structural optimization and design of microsatellite supporting legs// Axel SV, Sierk FB, Kurt M .Proceeding of World Congress of Structural and Multidisciplinary Optimization
Advanced structural topology optimization and application. ASMDO conference, Paris,
Application of a bi-level scheme including topology optimization to the design of an aircraft pylon
In this paper, topology optimization is used to design aircraft pylons. Original results for two Airbus pylons are first presented. An innovative bi-level optimization scheme is then proposed, which combines topology and geometric optimizations. At the first level, the dimension of the design domain, that is the envelope of the structure, and the location of the fixations are variables. At the second level, topology optimization is used to determine the optimal lay-out for given geometric parameters. This bi-level scheme is used to solve the aero-structural optimization of a pylon.
Structure sizing optimization capabilities at AIRBUS//Axel SV, Sierk FB, Kurt M. Proceeding of World Congress of Structural and Multidisciplinary Optimisation
Topology optimization of an additive layer manufactured (ALM) aerospace part.
Application of topology, sizing and shape optimization methods to optimal design of aircraft components. Altair Engineering Ltd. Proceeding of 3rd Altair UK HyperWorks Users Conference
Topology optimization of large scale turbine engine bracket assembly with additive manufacturing considerations// Axel SV, Sierk FB, Kurt M. Proceeding of World Congress of Structural and Multidisciplinary Optimization
Altair Engineering Inc
.,
Method for lightweight optimization for aerospace milled parts-case study for a helicopter pilot lightweight crashworthy seat side struts
Optimization assisted design of military transport aircraft structures. 10th AIAA/ISSMO Multidisciplinary Analysis and Optimization Conference, Albany,
Giga-voxel computational morphogenesis for structural design
Topology optimization of a blended-wing-body aircraft structure. 17th AIAA/ISSMO Multidisciplinary Analysis and Optimization Conference, Washington,
A new procedure for aerodynamic missile designs using topological optimization approach of continuum structures
<h2 class="secHeading" id="section_abstract">Abstract</h2><p id="">This paper presents a multi-objective programming scheme for the conceptual design of aerodynamic missile's structure using topological optimization approaches, in which both the compliance and eigenfrequency are regarded as static and dynamic optimization objectives, respectively. During the conceptual design of the aerodynamic missile with multiple loadings, both the multilevel sequential programming approach and the compromising programming method are coupled together to settle the associated difficulties when the whole structure of the missile body is considered as a pre-defined design domain. The compromise programming method is first applied to describe the statically loaded multi-stiffness topology optimization, and the dynamic formulation is used to establish the subsequent optimization problem mainly concerned with free vibration. The main advantage of the proposed scheme is the flexibility of dealing with optimal topology designs for the whole structures of aerodynamic missiles with complicated loading cases. Solid isotropic material with penalization (SIMP) is used as the interpolation scheme to indicate the dependence of material modulus upon regularized element densities. The sequential convex programming approach is applied to solve the optimization problem. An engineering application is used to demonstrate the characteristics of the presented methodologies based on the commercial software package of Hyperworks.OptiStruct at Altair®.</p>
Topology optimization with shape preserving design. The 5th International Conference on Computational Methods, Cambridge,
Conceptual design of aircraft structure based on topology optimization method//Axel SV, Sierk FB, Kurt M. Proceeding of World Congress of Structural and Multidisciplinary Optimisation
/
〈 |
|
〉 |
